Introduction
Quantum teleportation, once a concept confined to science fiction, is now a tangible reality in quantum mechanics and quantum information science. Unlike classical teleportation, which imagines the physical transport of objects, quantum teleportation involves the instantaneous transfer of quantum states between particles, utilizing the fundamental principles of quantum entanglement. This technology forms the backbone of future quantum communication networks, secure information transfer, and even potential advances in quantum computing.
Theoretical Foundations of Quantum Teleportation
At its core, quantum teleportation exploits quantum entanglement and Bell-state measurement to transmit quantum information. Consider two entangled particles, A and B, shared between two parties, Alice and Bob. When Alice wants to send an unknown quantum state from a third particle, C, to Bob, she performs a Bell-state measurement on particles A and C, collapsing their states into one of four possible entangled states. This process changes the state of Bob’s entangled particle B, such that it now contains the quantum information from C, but in an encoded form. Once Alice communicates her measurement results via a classical channel, Bob can apply the appropriate unitary transformation to recover the original quantum state of C in his particle B.
Quantum Circuit for Teleportation
The teleportation process can be represented as a quantum circuit:
Explanation of the Circuit
- Entanglement Generation: The first two qubits (Alice’s and Bob’s) are entangled using a Hadamard (H) gate followed by a CNOT gate.
- Bell Measurement: Alice applies a CNOT gate between her unknown quantum state and her entangled qubit, followed by a Hadamard gate and measurement.
- Classical Communication: Alice sends the classical measurement results (two bits) to Bob.
- State Recovery: Bob applies conditional unitary transformations (Pauli X and Z gates) to retrieve the teleported state.
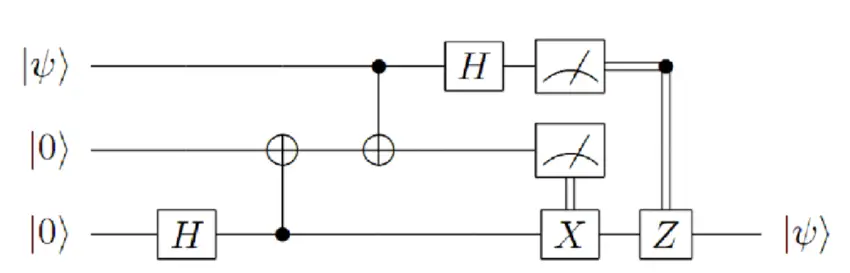
Key Components Enabling Quantum Teleportation
- Quantum Entanglement: The pre-existing correlation between particles that enables instant state transfer.
- Bell-State Measurement: A quantum measurement that projects entangled particles into one of four Bell states.
- Classical Communication Channel: Since quantum states cannot be cloned, classical information transfer is needed to complete the teleportation process.
- Quantum Gates for Correction: Bob applies quantum operations to reconstruct the original state based on Alice’s measurements.
Experimental Demonstrations & Breakthroughs
Quantum teleportation has been experimentally demonstrated in various settings:
- Photonic Quantum Teleportation: In 1997, the first successful quantum teleportation of a photonic qubit was demonstrated.
- Teleportation over Long Distances: In 2017, Chinese researchers achieved quantum teleportation of photons over 1,200 km using the Micius quantum satellite, proving the feasibility of quantum communication across vast distances.
- Teleportation of Matter Qubits: Researchers have extended teleportation beyond photons to trapped ions and superconducting qubits, crucial for quantum computing applications.
Applications of Quantum Teleportation
1. Secure Quantum Communication
Quantum teleportation is a key component in quantum cryptography, particularly in quantum key distribution (QKD), ensuring ultra-secure data transfer resistant to hacking or eavesdropping.
2. Quantum Repeaters for Quantum Internet
Quantum teleportation enables the creation of quantum repeaters, essential for building a quantum internet that allows secure, long-distance quantum communication using entanglement swapping.
3. Quantum Computing and Error Correction
In quantum computing, teleportation plays a critical role in linking qubits in distributed quantum systems and implementing fault-tolerant quantum circuits through teleported quantum gates.
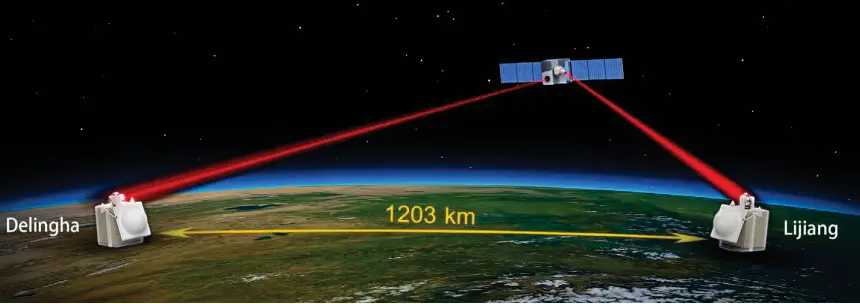
Challenges & Future Directions
While quantum teleportation has seen impressive
advancements, several challenges remain:
- Scalability
Issues: Large-scale entanglement distribution is needed for widespread
applications.
- Quantum
Memory and Storage: Efficient storage and retrieval of quantum states
is still an experimental hurdle.
- Decoherence
& Noise: Quantum systems are fragile and susceptible to
environmental disturbances.
Conclusion & Looking Ahead
Quantum teleportation is not just a theoretical marvel but
an evolving technology shaping the future of quantum communication,
cryptography, and computation. As researchers push the boundaries of
long-distance teleportation and entanglement-based technologies, the
realization of a fully functional quantum internet and next-generation quantum
computing architectures becomes increasingly feasible.
This article is part of a series on the Second Quantum
Revolution. The next installment will explore Quantum Cryptography &
Secure Communication, detailing how quantum principles can create virtually
unbreakable encryption methods.
Join us at OASIS 2025, where IZAK Scientific will
showcase innovations in quantum sensing and photonics.
At IZAK Scientific, we specialize in custom quantum
sensing solutions, helping industries harness the power of quantum technology.
References & Further Reading
- Bennett,
C. H., Brassard, G., Crépeau, C., Jozsa, R., Peres, A., & Wootters, W.
K. (1993). “Teleporting an unknown quantum state via dual classical
and Einstein-Podolsky-Rosen channels.” Physical Review Letters, 70(13),
1895-1899.
- Bouwmeester,
D., Pan, J. W., Mattle, K., Eibl, M., Weinfurter, H., & Zeilinger, A.
(1997). “Experimental quantum teleportation.” Nature, 390(6660),
575-579.
- Yin,
J., Cao, Y., Li, Y.-H., Liao, S.-K., Zhang, L., Ren, J.-G., … & Pan,
J.-W. (2017). “Satellite-based entanglement distribution over 1200
kilometers.” Science, 356(6343), 1140-1144.
- Pirandola,
S., Andersen, U. L., Banchi, L., Berta, M., Bunandar, D., Colbeck, R., …
& Walmsley, I. A. (2020). “Advances in quantum
cryptography.” Advances in Optics and Photonics, 12(4),
1012-1236.
- Gottesman,
D., & Chuang, I. L. (1999). “Demonstrating the viability of
universal quantum computation using teleportation and single-qubit
operations.” Nature, 402(6760), 390-393.
Tzachi Sabati
CEO, IZAK Scientific
Physicist specializing in photonics and quantum technologies, with deep expertise in quantum sensors and advanced optical systems. Leads the Advanced Quantum Lab course at the Technion, bridging academic excellence with industry innovation. At IZAK Scientific, provides cutting-edge photonics-based solutions, developing customized inspection and sensing systems for R&D and production. Passionate about advancing quantum sensing applications and integrating novel technologies to meet industry needs.