Innovations
A xenon flash lamp is a type of gas discharge lamp that produces intense white light for short durations. The physics behind its operation involves several key principles of gas discharge, photon emission, and electrical circuitry. Here’s a breakdown of how a xenon flash lamp works:
1. Construction and Components
A xenon flash lamp consists of a sealed glass tube filled with xenon gas at low pressure. Inside the tube, two electrodes are positioned at either end. The lamp is part of an electrical circuit that includes a power source as well as capacitor storage. The capacitor stores electrical energy that will be rapidly discharged through the xenon gas in the lamp.
2. Ionization and Electrical Discharge:
Electrical discharge is triggered and initiated by a high-voltage trigger pulse that momentarily ionizes the gas, making it electrically conductive and creating a path for the discharge.
3. Excitation of Xenon Atoms:
The electrical discharge through the xenon gas excites xenon atoms by knocking electrons to higher energy levels. When these electrons return to their original energy levels, the xenon atoms emit photons. The rapid discharge ensures that a significant number of xenon atoms are excited simultaneously, leading to the emission of a burst of light.
4. Light Emission:
The photons emitted by the excited xenon atoms cover a broad spectrum, from ultraviolet to visible to near-infrared light, producing a bright, white flash. The duration of the flash is typically in the order of microseconds to milliseconds, depending on the specific design of the flash lamp and the electrical characteristics of the circuit.
5. Cooling and Reset:
After the flash, the xenon gas quickly returns to its original state, and the lamp cools down. The storage capacitor is then recharged, preparing the flash lamp for the next operation.
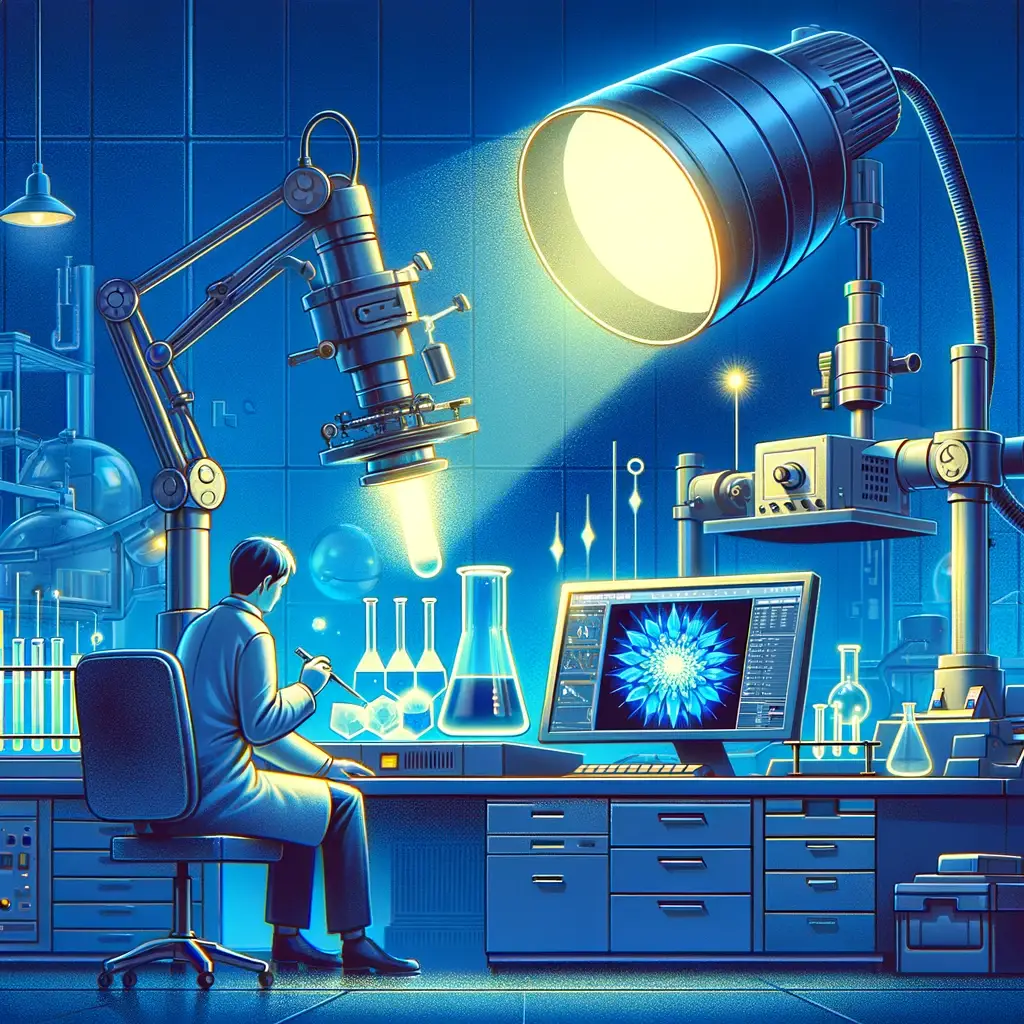
Innovations
A xenon flash lamp is a type of gas discharge lamp that produces intense white light for short durations. The physics behind its operation involves several key principles of gas discharge, photon emission, and electrical circuitry. Here’s a breakdown of how a xenon flash lamp works:
1. Construction and Components
A xenon flash lamp consists of a sealed glass tube filled with xenon gas at low pressure. Inside the tube, two electrodes are positioned at either end. The lamp is part of an electrical circuit that includes a power source as well as capacitor storage. The capacitor stores electrical energy that will be rapidly discharged through the xenon gas in the lamp.
2. Ionization and Electrical Discharge:
Electrical discharge is triggered and initiated by a high-voltage trigger pulse that momentarily ionizes the gas, making it electrically conductive and creating a path for the discharge.
3. Excitation of Xenon Atoms:
The electrical discharge through the xenon gas excites xenon atoms by knocking electrons to higher energy levels. When these electrons return to their original energy levels, the xenon atoms emit photons. The rapid discharge ensures that a significant number of xenon atoms are excited simultaneously, leading to the emission of a burst of light.
4. Light Emission:
The photons emitted by the excited xenon atoms cover a broad spectrum, from ultraviolet to visible to near-infrared light, producing a bright, white flash. The duration of the flash is typically in the order of microseconds to milliseconds, depending on the specific design of the flash lamp and the electrical characteristics of the circuit.
5. Cooling and Reset:
After the flash, the xenon gas quickly returns to its original state, and the lamp cools down. The storage capacitor is then recharged, preparing the flash lamp for the next operation.
Key Features of Xenon Flash Lamps:
Intensity and Broad Spectrum: Xenon flash lamps can produce extremely bright light that spans a wide spectral range, making them suitable for various applications, including photography, scientific research, and industrial processes.
Pulse Duration: The duration of the light pulse can be precisely controlled through the design of the electrical circuit and the characteristics of the xenon gas and lamp construction.
Repeatability: Xenon flash lamps can be designed to operate at different repetition rates, from single flashes to several hundred flashes per second, depending on the application requirements.
The operation of xenon flash lamps beautifully demonstrates the principles of gas discharge physics, the interaction of electric fields with matter, and the conversion of electrical energy into light. This makes them suitable for a wide range of interesting measurements and applications in both research and industry. Here are some notable examples:
Photoluminescence Spectroscopy:
Measurement: Photoluminescence (PL) spectroscopy involves exciting a sample with a light source and measuring the light it subsequently emits. A xenon flash lamp can provide the high-intensity, broad-spectrum light necessary to excite a wide range of materials.
Application: This technique is widely used in the study of semiconductor materials, quantum dots, and other photonic structures to understand their electronic and optical properties.
Time-Resolved Spectroscopy:
Measurement: Time-resolved spectroscopy tracks changes in the properties of a sample over time after it has been excited by a light pulse. Xenon flash lamps can generate short, intense light pulses suitable for initiating rapid photophysical or photochemical processes.
Application: This method is essential in studying fast processes such as fluorescence lifetime measurements, energy transfer mechanisms, and chemical reaction dynamics.
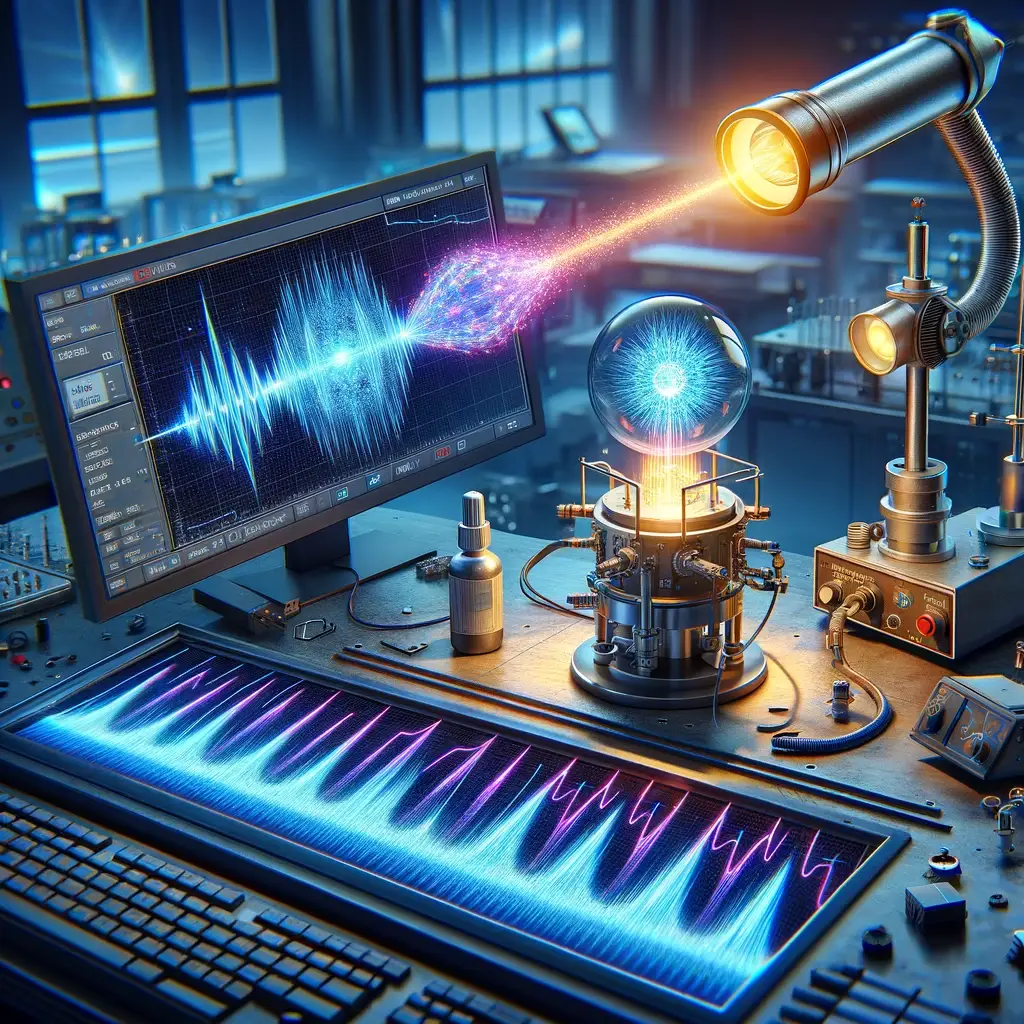
UV-Vis Absorption Spectroscopy:
Measurement: Ultraviolet-visible (UV-Vis) spectroscopy measures the absorption of UV or visible light by a sample. A xenon flash lamp’s broad spectrum allows for comprehensive absorption measurements across a wide range of wavelengths.
Application: This technique is crucial in analyzing the concentration and purity of substances, characterizing materials, and studying electronic transitions in molecules.
Flash Photolysis:
Measurement: Flash photolysis is a technique where a brief intense light pulse from a xenon flash lamp is used to dissociate molecules in a sample, creating reactive species. The transient species produced are then studied using spectroscopic methods.
Application: Flash photolysis is used to investigate the mechanisms of fast photochemical reactions, including the study of reaction intermediates and kinetics.
Solar Simulator:
Measurement: Xenon flash lamps can simulate sunlight conditions by providing a continuous spectrum that closely matches natural sunlight. This allows for the measurement of photovoltaic cell efficiency under standardized conditions.
Application: Solar simulators are used in the development and testing of solar cells and solar thermal devices, enabling researchers and manufacturers to optimize their performance and stability.
Optical Pumping and Laser Studies:
Measurement: Optical pumping uses light to raise atoms or molecules from a lower energy state to a higher energy state. Xenon flash lamps can serve as pump sources for certain types of lasers or for studying the optical properties of materials.
Application: This technique is important for initializing population inversion in laser media, studying energy transfer processes, and investigating the optical properties of new laser gain materials.
Photodynamic Therapy (PDT) Research:
Measurement: In PDT research, light-sensitive compounds are activated using light of specific wavelengths to produce reactive oxygen species that can kill cancer cells. Xenon flash lamps can provide the broad-spectrum or tunable light source required to activate these compounds.
Application: This application is critical in the development and optimization of photodynamic therapy treatments for cancer and other diseases.
These measurements highlight the versatility of xenon flash lamps in facilitating a broad spectrum of scientific and industrial applications, thanks to their ability to deliver high-intensity, broad-spectrum, and pulsed light.
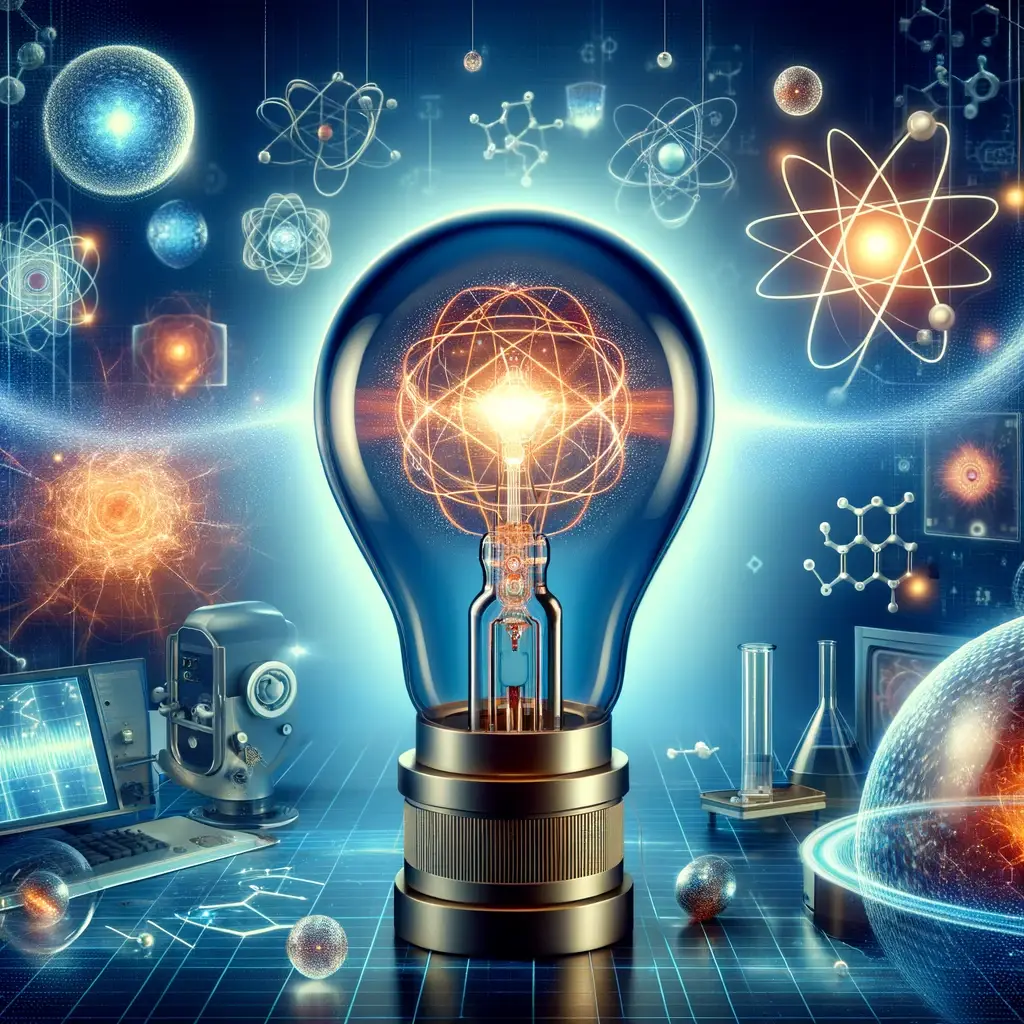
Quantum Applications for Xenon flash lamp
Quantum research leveraging xenon lamps or xenon flash lamps spans a diverse array of studies, emphasizing the versatility and utility of these light sources in advancing our understanding of quantum mechanics and its applications. Here are some examples of quantum researches using xenon lamps or xenon flash lamps:
Thermally Assisted Flash Annealing of Silicon and Germanium: Investigates the use of radiant energy from high-intensity xenon flash lamps to anneal damage in semiconductors, a process critical for the development of quantum computing components and sensors (Cohen et al., 1978).
Mean Quantum Yields for Photolysis of Benzophenones in Solution: Explores the photochemical stability of compounds exposed to broad-band radiation with a high photon density from xenon flash lamps, fundamental for understanding photoinduced quantum processes (Lebedeva et al., 1979).
Xenon Flash Lamp Annealing of Poly-Si Thin Films: Discusses xenon flash lamp annealing as a method for crystallizing amorphous silicon films, crucial for fabricating quantum dots and other nanostructures for quantum computing (Terai et al., 2006).
Rapid Photonic Processing of High-Electron-Mobility PbS Colloidal Quantum Dot Transistors: Utilizes xenon flash lamp sintering to post-treat solution-deposited layers of lead sulfide quantum dots, a key technique for developing quantum dot-based electronic devices (Nugraha et al., 2020).
In Vivo Imaging of Quantum Dots Encapsulated in Phospholipid Micelles: Describes the use of fluorescent semiconductor nanocrystals (quantum dots) for biological imaging, highlighting the potential of xenon flash lamps in excitation sources for quantum dot studies (Dubertret et al., 2002).
These studies showcase the crucial role of xenon flash lamps in quantum research, from materials science and photonic processing to biological imaging and the development of quantum computing technologies.
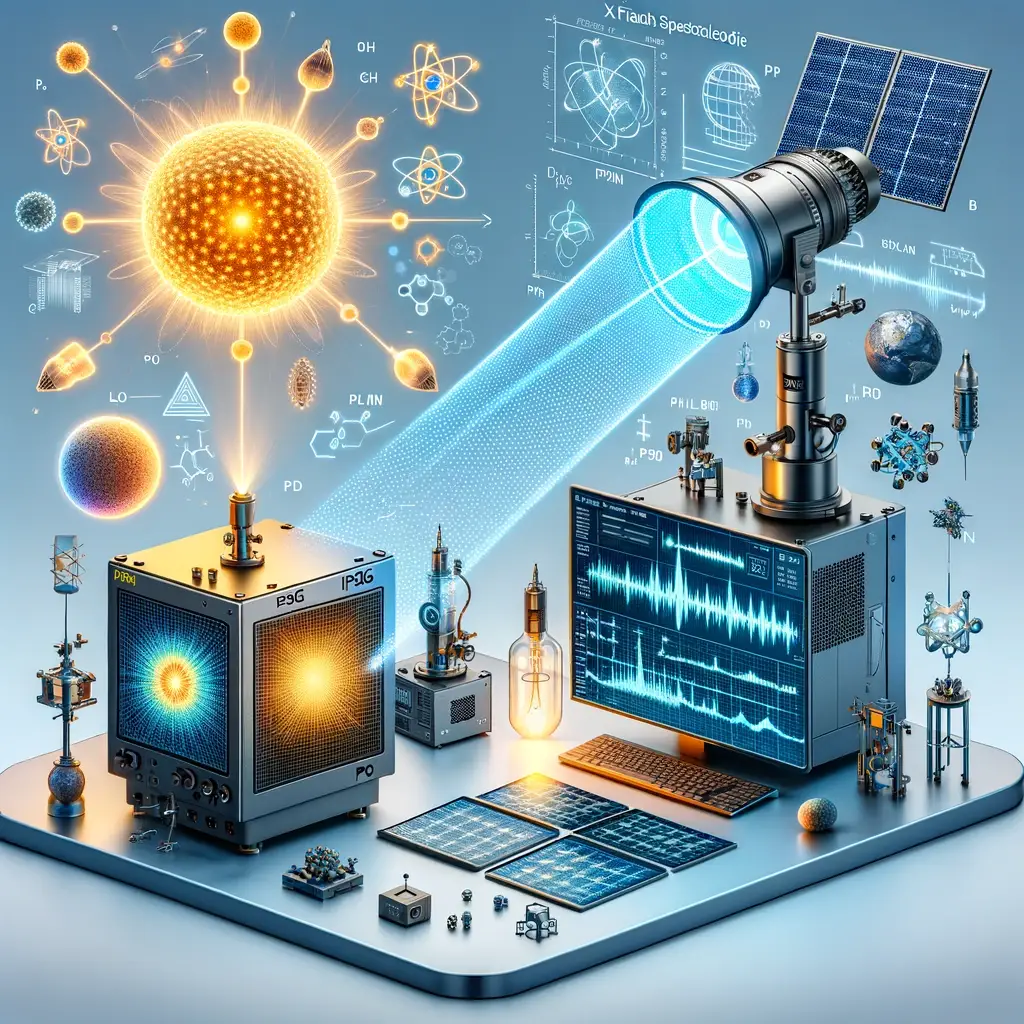
In the realm of scientific research and technological applications, Xenon flash lamps stand out as versatile and powerful tools, offering a broad spectrum of light that spans from ultraviolet (UV) through visible to infrared (IR) wavelengths. These capabilities make Xenon flash lamps indispensable in a wide range of applications, from photoluminescence spectroscopy to solar simulation and quantum technology applications. Two systems that exemplify the advancement in Xenon flash lamp technology are the PSNG and PXL systems developed by IZAK Scientific.
The PSNG System: A Leap in Low Power Xenon Lamp Technology
The PSNG system is tailored for applications requiring low power Xenon lamps, capable of operating up to 3Hz with an operation voltage of up to 1kV, alongside an ignition channel ranging from 5kV to 10kV. This system is an ideal solution for applications such as Photoluminescence Spectroscopy, where precision and controlled light output are paramount. The PSNG system’s design ensures reliability and consistency in experiments that necessitate lower intensity but high-quality light sources.
The PXL System: Powering High-Intensity Applications
On the other end of the spectrum, the PXL system is designed for high-power applications, boasting a repetition rate capability of up to 100Hz. The lamp operation voltage can range from 200V to 4kV, with an ignition level spanning from 5kV to 20kV or more. This robust system can drive up to 5 lamps simultaneously, achieving a total of 100Hz in flash repetition rate. The PXL system is perfectly suited for high-intensity applications such as Solar Simulators, where simulating the full intensity and spectrum of sunlight is crucial for testing photovoltaic materials and solar cells.
Integrating PSNG and PXL Systems into Scientific Research
The versatility of the PSNG and PXL systems by IZAK Scientific opens up a new horizon of possibilities for researchers and technologists. For instance, in Photoluminescence Spectroscopy, the PSNG system’s low power and high precision capabilities enable the detailed analysis of semiconductor materials and quantum dots, unlocking insights into their electronic and optical properties. Meanwhile, the PXL system‘s high power and fast repetition rate make it an indispensable tool in Solar Simulator applications, aiding in the development and testing of solar cells by providing a consistent and controllable artificial sunlight environment.
A notable addition to their application spectrum is their use as intense flash lamps for camera shooting, illuminating dark backgrounds, and serving as illuminators for imaging testers of vehicle tires.
Intense Flash Lamp for Camera Shooting
The adaptability of the PSNG and PXL systems extends into the realm of photography and videography, where the need for high-quality, intense lighting is paramount. These systems can be harnessed as powerful flash lamps to brighten dark scenes or create dramatic lighting effects, ensuring that every shot captures the fullest detail and color. The ability to control the intensity and repetition rate of the flash allows photographers and videographers to achieve the perfect illumination for their subjects, making these systems invaluable tools in professional and creative settings.
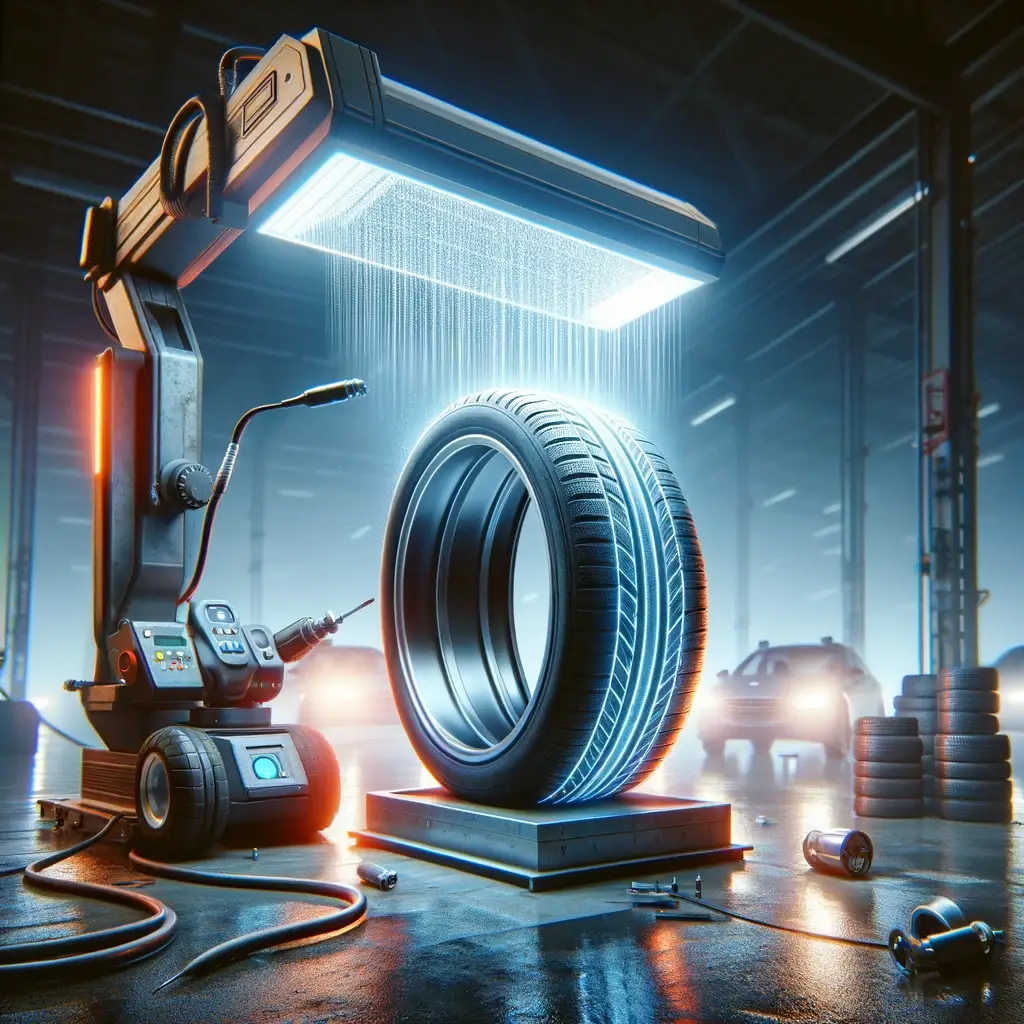
Illuminator for Imaging Tester of Vehicle Tires
In the automotive industry, the safety and reliability of vehicle tires are of utmost importance. The PXL system, with its high-power capabilities, provides an ideal solution for tire inspection processes. As an illuminator for imaging testers, it can reveal minute defects and inconsistencies in tire materials and construction that might otherwise remain undetected. The intense and precise illumination ensures that every aspect of the tire is thoroughly examined, contributing to safer and more reliable automotive products.
Conclusions
The integration of the PSNG and PXL systems into areas such as photography and automotive safety testing illustrates the vast potential of Xenon flash lamp technology beyond traditional scientific research. Whether it’s capturing the perfect photograph in challenging lighting conditions or ensuring the integrity of vehicle tires, these systems offer the high performance, versatility, and reliability needed to excel in diverse applications. As we continue to explore the capabilities of the PSNG and PXL systems, their contribution to both science and industry becomes increasingly invaluable, demonstrating the enduring relevance and adaptability of Xenon flash lamp technology.
The PSNG and PXL systems represent the cutting edge of Xenon flash lamp technology, offering solutions tailored to both low and high power applications. Whether it’s conducting delicate spectroscopy experiments or simulating the harsh conditions of sunlight, these systems provide the reliability, versatility, and precision that modern scientific research and technological development demand. Their integration into various scientific and industrial applications highlights the pivotal role of advanced Xenon flash lamp systems in pushing the boundaries of what’s possible in research and development.
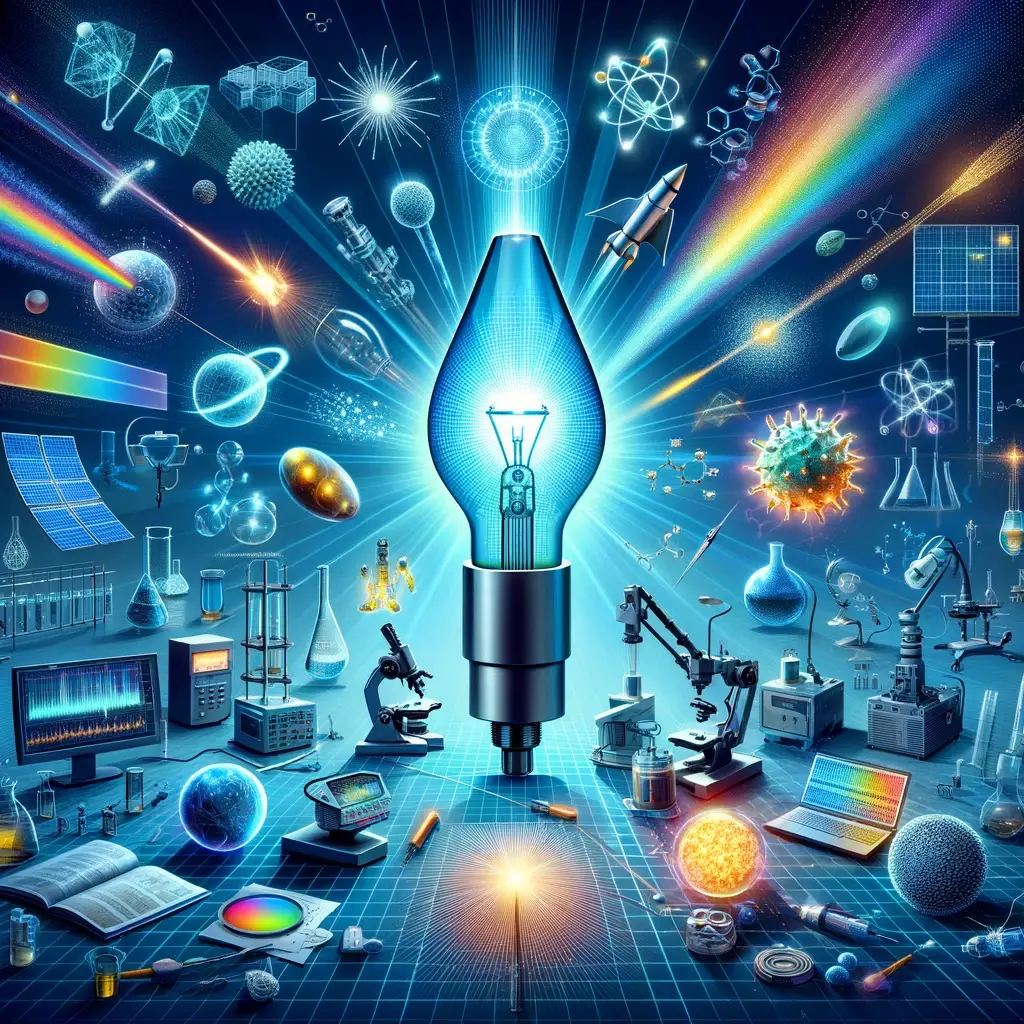
References:
For the article discussing various measurements that can be carried out with a xenon flash lamp, here is a reference list providing foundational and applied research insights into the mentioned techniques and applications:
1. Ikeda, H., Namai, H., & Hirano, T. (2005). Spectroscopic and DFT evidence for a nonclassical radical cation derived from 7-benzhydrylidenenorbornene. Tetrahedron Letters, 46, 3917-3921.
2. Yasui, S., Kobayashi, S., & Mishima, M. (2016). Kinetic study of photoreaction of triarylphosphines by laser flash photolysis time-resolved UV-VIS spectroscopy. Phosphorus, Sulfur, and Silicon and the Related Elements, 191, 1-3.
3. Johnson, J. C., Knutsen, K., Yan, H., Law, M., Zhang, Y., & Saykally, R. (2004). Ultrafast Carrier Dynamics in Single ZnO Nanowire and Nanoribbon Lasers. Nano Letters, 4, 197-204.
4. Oyama, M., Okazaki, S., & Nagamura, T. (1991). Measurement of time-resolved absorption spectra of species generated by fast electrochemical processes. Analytica Chimica Acta, 245, 199-202.
5. Younesi, A. T., & Ulbricht, R. (2022). Broadband transient absorption spectroscopy using an incoherent white-light source as probe. Optics Express, 30 21, 38896-38906
6. Fasol, G. (1982). Time-resolved photoluminescence measurements using a boxcar averager. Journal of Physics E: Scientific Instruments, 15, 1296-1299.
7. Zhang, X., Yu, L., Zhuang, C., Peng, T., Li, R., & Li, X. (2014). Highly Asymmetric Phthalocyanine as a Sensitizer of Graphitic Carbon Nitride for Extremely Efficient Photocatalytic H2 Production under Near-Infrared Light. ACS Catalysis, 4, 162-170.
8. Barugkin, C., Cong, J., Duong, T., Rahman, S., Nguyen, H. T., Macdonald, D., White, T., & Catchpole, K. (2015). Ultralow Absorption Coefficient and Temperature Dependence of Radiative Recombination of CH3NH3PbI3 Perovskite from Photoluminescence. The Journal of Physical Chemistry Letters, 6 5, 767-72.
9. Cohen, R. L., Williams, J., Feldman, L., & West, K. (1978). Thermally assisted flash annealing of silicon and germanium. Applied Physics Letters, 33, 751-753.
10. Lebedeva, E. P., Matyushin, G. A., Mezentseva, G. A., Tavrizova, M. A., & Fain, V. Y. (1979). Mean quantum yields for photolysis of benzophenones in solution when illuminated with a flash lamp. Journal of Applied Spectroscopy, 31, 884-887.
11. Terai, F., Matunaka, S., Akihiko, T., Ichimura, C., Nagatomo, T., & Homma, T. (2006). Xenon Flash Lamp Annealing of Poly-Si Thin Films. Journal of The Electrochemical Society.
12. Nugraha, M., Yarali, E., Firdaus, Y., Lin, Y., El‐Labban, A., Gedda, M., Lidorikis, E., Yengel, E., Faber, H., & Anthopoulos, T. (2020). Rapid Photonic Processing of High-Electron-Mobility PbS Colloidal Quantum Dot Transistors. ACS Applied Materials & Interfaces, 12, 31591-31600.
13. Dubertret, B., Skourides, P., Norris, D. J., Noireaux, V., Brivanlou, A. H., & Libchaber, A. (2002). In Vivo Imaging of Quantum Dots Encapsulated in Phospholipid Micelles. Science, 298, 1759-1762.
These references span a variety of applications and studies involving xenon flash lamps, from photoreactions and carrier dynamics in nanomaterials to advanced spectroscopic techniques and photoluminescence studies, demonstrating the broad utility of xenon flash lamps in scientific research as well as in quantum research.
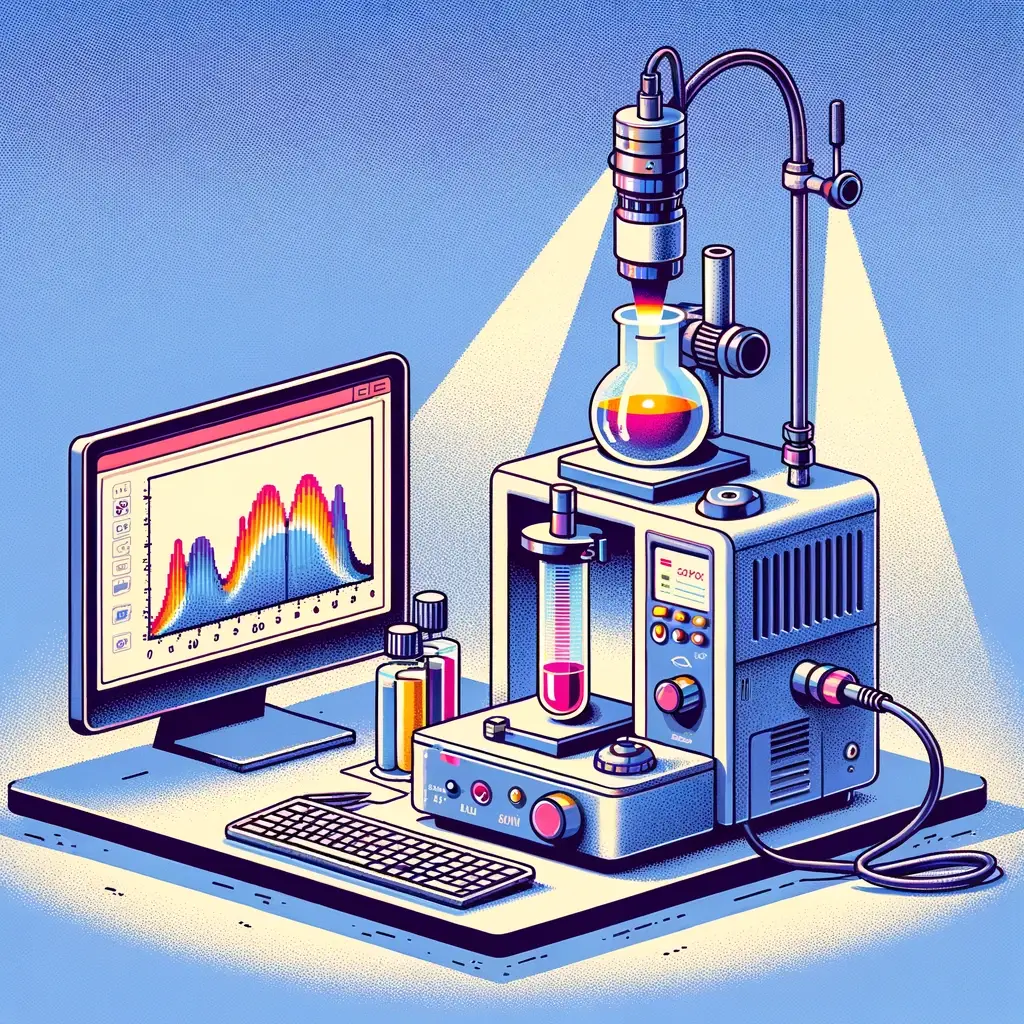