In today’s fast-paced digital world, the demand for high-speed, reliable communication has never been greater. At the heart of this technological revolution lies a surprisingly thin and flexible medium: optical fiber. This beginner’s guide will demystify fiber optics, explaining its principles, benefits, and wide-ranging applications.
What is Fiber Optics?
Fiber optics is the technology associated with the transmission of information as light pulses along a glass or plastic strand or fiber. Unlike traditional metal wires that transmit electricity, fiber optic cables transmit light, making them capable of delivering higher bandwidth over longer distances with minimal loss of signal.
How Does it Work?
The core principle behind fiber optics is total internal reflection. Each optical fiber consists of a core and a cladding layer. The core, made from glass or plastic, carries the light, while the cladding, with a lower refractive index, traps the light within the core through reflection. This allows light to travel through the fiber over great distances with little loss.
Understanding the Science Behind Optical Fibers
To appreciate the technological marvel that is optical fiber, it’s essential to delve into the physics underpinning its operation. This section aims to elevate your understanding by exploring the fundamental scientific principles such as total internal reflection, numerical aperture, bending radius, and other critical properties that make optical fibers the backbone of modern communication.
Total Internal Reflection
At the heart of optical fiber technology is the phenomenon known as total internal reflection. This physical principle allows light to be guided along the fiber over long distances with minimal loss. When light travels within the core of an optical fiber and hits the boundary with the cladding at a sufficiently shallow angle, it is reflected back into the core rather than passing into the cladding. This occurs because the core’s material has a higher refractive index than the cladding, creating a condition where the light is completely reflected back into the core if the incidence angle is above a certain critical angle. This ensures that light can travel through the fiber with minimal attenuation, making long-distance light transmission feasible.
Numerical Aperture
The numerical aperture (NA) of an optical fiber is a dimensionless number that characterizes its ability to gather light. It is defined by the sine of the maximum angle of light that can enter the fiber and still be totally internally reflected. The NA is a function of the refractive indices of the core and the cladding:
NA= sqrt(ncore² – ncladding² )
Where ncore and ncladding are the refractive indices of the core and cladding, respectively. A higher NA means the fiber can accept light at a wider range of angles, which is particularly useful in applications requiring efficient light coupling from a light source into the fiber.
Bending Radius
The bending radius of an optical fiber refers to the minimum radius a fiber can be bent without significantly impairing its performance. Bending the fiber too tightly can cause light to escape from the core into the cladding, leading to increased signal loss. This property is crucial in the installation and handling of fiber optic cables, as it dictates how they can be routed and manipulated within various environments without compromising their integrity.
Other Properties
Attenuation: This is the reduction in signal strength as light travels through the fiber, measured in decibels per kilometer (dB/km). Factors contributing to attenuation include scattering, absorption, and bending losses.
Dispersion: This phenomenon refers to the spreading of light pulses over time as they travel through the fiber, which can lead to signal degradation. Dispersion arises from the different speeds at which various wavelengths of light travel through the fiber.
Bandwidth: This property describes the capacity of the fiber to carry information, often influenced by both attenuation and dispersion. Advances in fiber design are continuously improving bandwidth capabilities to meet the demands of burgeoning data transmission needs.
The principles of total internal reflection, numerical aperture, bending radius, and other optical properties are fundamental to the design and function of optical fibers. Understanding these scientific concepts not only enhances our appreciation of this technology but also informs the development of more advanced, efficient, and robust fiber optic systems. As we continue to push the boundaries of physics and materials science, the future of optical fiber technology holds even greater promise for transforming our connected world.
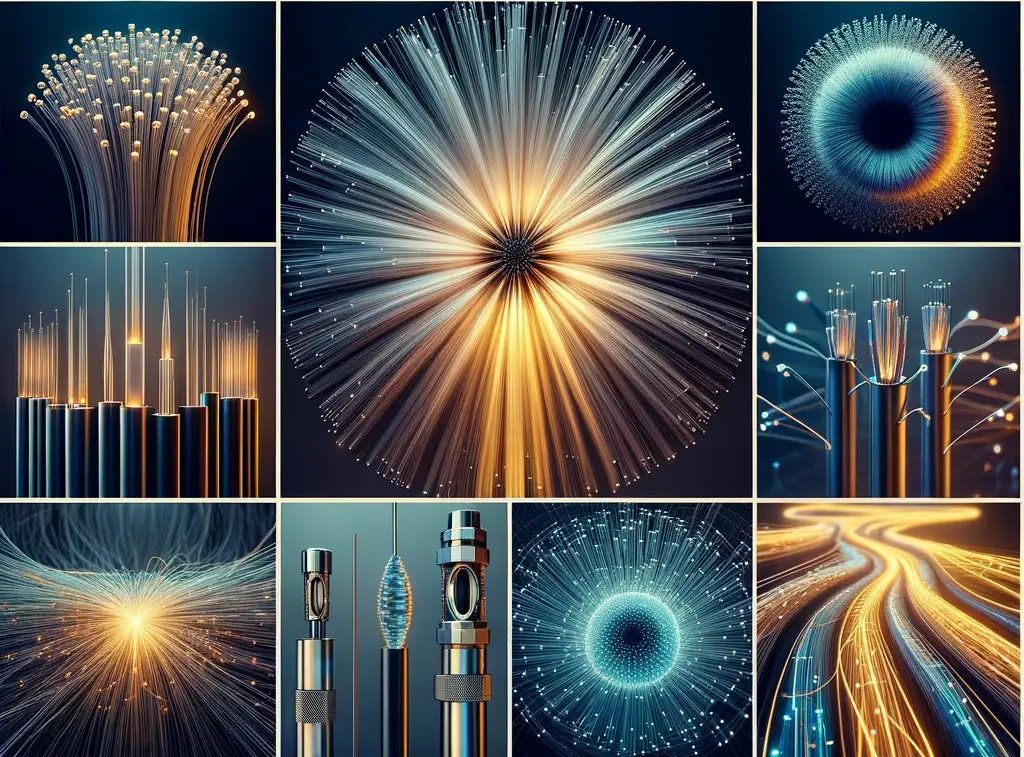
Understanding Low-OH and High-OH in Optical Fiber
Optical fibers are categorized based on their chemical composition and the presence of hydroxyl (OH) ions, which significantly influence their transmission properties, particularly in the infrared (IR) region. The terms “Low-OH” and “High-OH” refer to the concentration of hydroxyl ions within the fiber’s silica glass.
Low-OH Optical Fibers
Low-OH fibers have a reduced concentration of hydroxyl ions. This characteristic makes them highly suitable for applications requiring efficient transmission of light in the infrared (IR) spectrum. Low-OH fibers exhibit lower attenuation in the IR region, making them ideal for telecommunications and sensing applications that operate within these wavelengths. The reduction in hydroxyl ion content decreases the absorption peaks associated with OH ions, thus minimizing signal loss and enhancing the fiber’s performance for long-distance communication.
High-OH Optical Fibers
Conversely, High-OH fibers contain a higher concentration of hydroxyl ions. This composition increases the fiber’s ability to transmit light in the ultraviolet (UV) and visible spectrum but introduces higher attenuation in the IR region due to the absorption peaks of OH ions. High-OH fibers are particularly advantageous in applications requiring transmission of light at UV and visible wavelengths, such as medical devices, spectroscopy, and certain types of sensors and lasers.
The choice between Low-OH and High-OH fibers depends on the specific requirements of the application, including the operational wavelength and the environment in which the fiber will be used.
The distinction between single-mode and multi-mode fibers
These two types of optical fibers serve different purposes in telecommunications, data transmission, and various applications, based on their unique properties and the way they guide light.
Single-Mode Fiber (SMF)
Single-mode fiber is designed to carry light directly down the fiber, with minimal reflection and dispersion. It features a very small core, typically around 8 to 10 micrometers in diameter, which allows only one mode of light to propagate. This singular path prevents modal dispersion, a phenomenon where light rays travel at different speeds, causing signal distortion over long distances. As a result, single-mode fibers offer high bandwidth and can transmit data over much longer distances than multi-mode fibers, making them ideal for long-haul telecommunications, cable TV, and Internet backbone connections.
Multi-Mode Fiber (MMF)
Multi-mode fiber, on the other hand, has a larger core diameter, typically ranging from 50 micrometers up to 1mm or more. This larger size allows multiple modes or paths of light to propagate simultaneously. While this design enables the fiber to carry more light and support higher data rates over short distances, it also leads to modal dispersion, which limits the transmission distance and overall bandwidth compared to single-mode fibers.
Multi-mode fibers are commonly used in shorter-distance applications, such as within buildings or on campus networks, where high data rates are needed over relatively short distances.
Multimode fibers are extensively used in medical devices for the delivery of energy, particularly for therapeutic and diagnostic applications. Their ability to transmit light/energy over relatively short distances makes them ideal for a variety of medical uses, including surgery, imaging, and treatment delivery
Key Differences between single-mode fiber and multi-mode fiber
· Core Size: Single-mode fibers have a much smaller core than multi-mode fibers, allowing only one path for light to travel.
· Data Transmission: Single-mode fibers can transmit data over longer distances with higher bandwidth. Multi-mode fibers are suited for shorter distances but can support higher data rates at those distances.
· Applications: Single-mode fibers are used for long-distance telecommunications and high-speed data connections. Multi-mode fibers are preferred for short-range data communication within local area networks (LANs) and data centers.
In summary, the choice between single-mode and multi-mode fiber depends on the specific needs of the application, including the required transmission distance, data rate, and budget considerations. Understanding these differences is crucial for anyone looking to navigate the world of fiber optics, whether for professional use or general knowledge.
A Brief About Hollow Fiber
Hollow fiber optics, distinct from solid silica optical fibers, feature a central hollow core surrounded by a cladding layer. This unique structure allows light to propagate through the air or vacuum inside the core, relying on the principle of internal reflection at the core-cladding interface. Hollow fibers offer several advantages:
· Low Material Absorption: Since light travels primarily through air or vacuum, absorption by the fiber material is significantly reduced, making hollow fibers suitable for transmitting high-power laser beams and light at wavelengths that would be absorbed by solid fibers.
· High Damage Threshold: The air or vacuum core can withstand higher power densities than solid materials, reducing the risk of damage from high-intensity light sources.
· Flexible Transmission Windows: By adjusting the internal coating or structure, hollow fibers can be optimized for efficient transmission at specific wavelengths, including those challenging for solid fibers, such as far-IR or UV.
Hollow fiber optics are particularly valuable in applications requiring the transmission of high-power lasers, precise delivery of light in medical procedures, or transmission at wavelengths poorly suited to traditional solid fibers. Their development and implementation continue to expand the capabilities and applications of fiber optic technology.
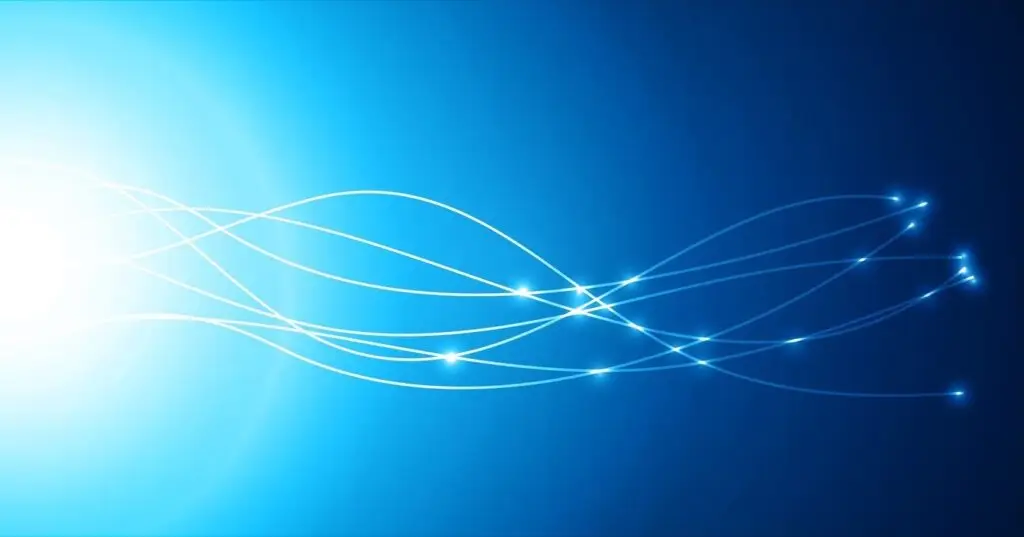
Components of Fiber Optic Systems
· Transmitter: Converts electronic signals into light signals.
· Optical Fiber: The medium through which the light signal travels.
· Amplifiers: Boost the light signal during its journey, ensuring it reaches the other end with sufficient strength.
· Receivers: Convert light signals back into electronic signals.
Benefits of Fiber Optics
· High Bandwidth: Optical fibers can carry a vast amount of data, far exceeding traditional copper cables.
· Long Distance Transmission: Signals in optical fibers degrade less over distance, allowing for long-range communication without significant loss of quality.
· Resistance to Electromagnetic Interference: Unlike copper cables, fiber optics are immune to interference from electromagnetic fields, ensuring clearer communication.
· Safety and Security: Optical fibers are safe from sparks and are difficult to tap into without detection, making them secure from data breaches.
Applications of Fiber Optics
· Telecommunications: Fiber optics form the backbone of internet, telephone, and cable television systems worldwide.
· Medical Devices: Used in a variety of instruments for imaging, surgical, and diagnostic applications.
· Industrial and Environmental Sensing: Fiber optics are used in sensors to measure temperature, pressure, and other parameters in harsh environments.
· Defense and Aerospace: For secure, reliable communication systems and in the manufacture of advanced aircraft and spacecraft.
· Home and Office Networks: Fiber optic cables are increasingly used for high-speed internet connections in homes and businesses.
The Future of Fiber Optics
The future of fiber optics is incredibly bright. With ongoing advancements in fiber optic technology, we’re seeing the development of even thinner, more flexible fibers with greater capacity. Innovations such as photonic crystal fibers (PCFs) are opening new avenues for data transmission and sensing applications.
Conclusion
Fiber optics is a fascinating and vital technology that has revolutionized the way we communicate, work, and entertain ourselves. Its ability to transmit vast amounts of data over long distances quickly and securely makes it a cornerstone of modern technology. As we continue to push the boundaries of what’s possible with fiber optics, we can expect even more innovative and life-changing applications to emerge.
Whether you’re a technology enthusiast keen to understand the latest advancements or someone curious about the science behind our connected world, the world of fiber optics offers endless avenues for exploration and discovery.
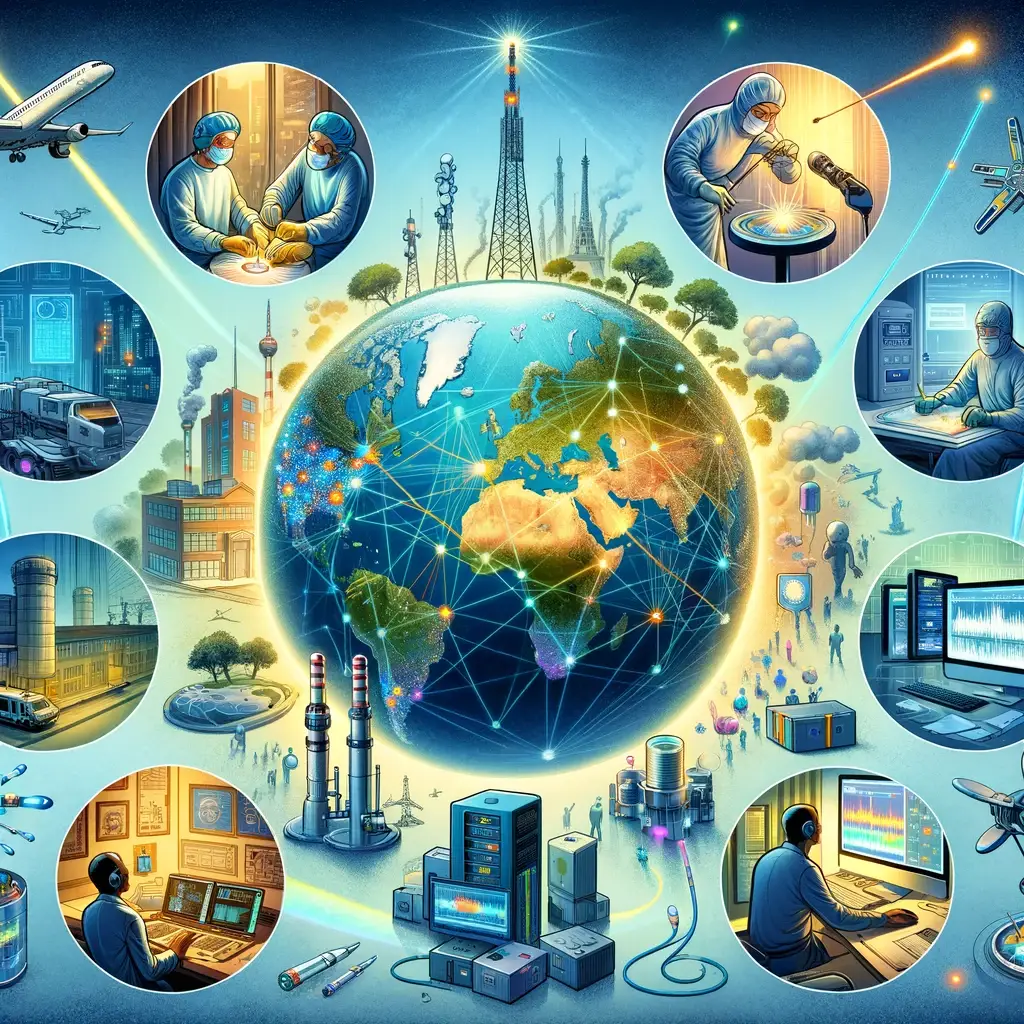
Fiber Testing Solutions by IZAK Scientific
In concluding our guide to fiber optics, the role of meticulous testing in maintaining the quality and efficiency of fiber optic networks is undeniable. IZAK Scientific contributes significantly to this field with its development of precision testing equipment tailored for various fiber optic applications.
At IZAK Scientific, we offer a suite of fiber testing tools designed to evaluate the performance of fiber optics, ensuring they meet industry standards and application-specific requirements. Our focus on customization allows us to create testing solutions that align with the unique needs of each client, covering a wide range of uses from telecommunications to industrial sensing.
Our commitment to innovation means each testing tool and system we provide is built to deliver accurate and dependable results, supporting the reliable deployment of fiber optic technologies. IZAK Scientific’s approach to testing emphasizes precision, adaptability, and quality, ensuring our clients can fully leverage the capabilities of fiber optics in their operations.
Through this guide, we’ve touched on the essentials of fiber optics, highlighting its applications, benefits, and the critical importance of thorough testing. As the fiber optics field evolves, IZAK Scientific remains dedicated to supporting its advancement with effective testing solutions.
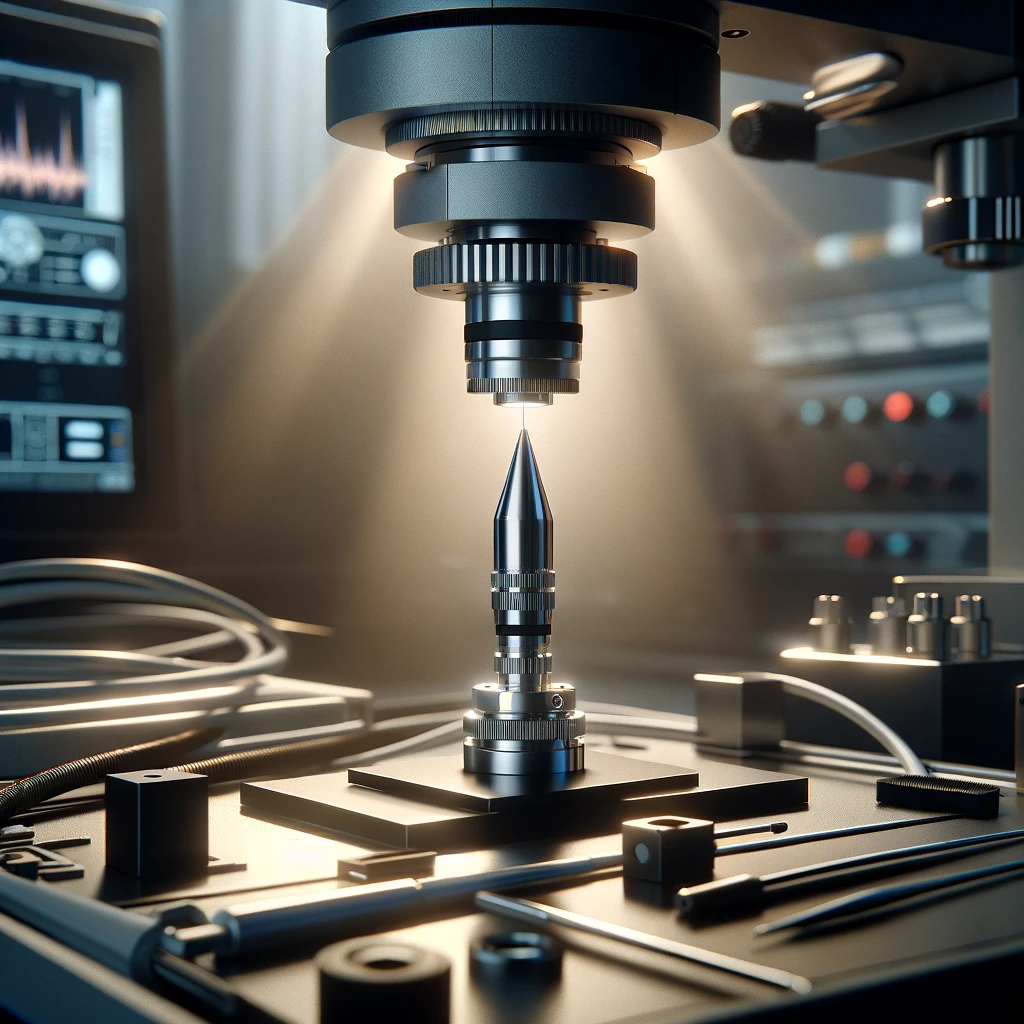
Reference
list
Involves
citing relevant literature that covers the principles and applications of fiber
optics. Here’s a reference list that includes foundational texts, research
articles, and resources on the subject:
1. Agrawal, G. P.
(2012). Fiber-Optic Communication Systems. Wiley. This book provides an
extensive overview of fiber-optic communication systems, including the physical
principles of fiber optics, system design considerations, and applications.
2. Hecht, J.
(2018). Understanding Fiber Optics. 6th Edition, Jeff Hecht. A comprehensive
guide for beginners and professionals alike, this book covers the basics of
fiber optics, its components, and its wide range of applications.
3. Senior, J. M.,
& Jamro, M. Y. (2009). Optical Fiber Communications: Principles and
Practice. 3rd Edition, Prentice Hall. This textbook introduces the fundamental
concepts of optical fiber technology, including the physics of fiber optics,
system design issues, and practical applications.
4. Snyder, A. W.,
& Love, J. (1983). Optical Waveguide Theory. Chapman and Hall. This book
provides a theoretical background on optical waveguides, which are essential
components of fiber optics, detailing waveguide properties, light transmission,
and modal analysis.
5. Keiser, G.
(2011). Optical Fiber Communications. 4th Edition, McGraw-Hill. This resource
covers the technological advancements in optical fiber communication systems,
including the role of optical fibers in modern telecommunications.
6. Marcuse, D.
(1977). Theory of Dielectric Optical Waveguides. Academic Press. This work
delves into the theoretical aspects of dielectric optical waveguides, a core
concept in understanding how optical fibers guide light.
7. Palais, J. C.
(2005). Fiber Optic Communications. 5th Edition, Prentice Hall. This text
explores the basics of fiber optic communications, including the history,
development, and future directions of fiber optic technology.
8. Ghatak, A.,
& Thyagarajan, K. (1998). Introduction to Fiber Optics. Cambridge
University Press. This book introduces the principles of fiber optics,
emphasizing the physical and engineering aspects of fiber optic communication
systems.
9. Rajan, G.
(2017). Fiber Optic Sensors: Fundamentals and Applications. SPIE Press. This
publication highlights the use of fiber optics in sensing applications,
detailing the principles, fabrication, and deployment of fiber optic sensors.
10. Kersey, A. D.,
Davis, M. A., Patrick, H. J., LeBlanc, M., Koo, K. P., Askins, C. G., Putnam,
M. A., & Friebele, E. J. (1997). Fiber grating sensors. Journal of
Lightwave Technology, 15(8), 1442-1463. This article discusses the application
of fiber gratings as sensors, showcasing the versatility of fiber optics beyond
telecommunications.
These
references provide a foundation for understanding the multifaceted world of
fiber optics, from basic principles to cutting-edge applications. Whether for
educational purposes or professional development, these resources offer
valuable insights into the science and technology of fiber optics.
Tzachi Sabati
CEO, IZAK Scientific
Physicist specializing in photonics and quantum technologies, with deep expertise in quantum sensors and advanced optical systems. Leads the Advanced Quantum Lab course at the Technion, bridging academic excellence with industry innovation. At IZAK Scientific, provides cutting-edge photonics-based solutions, developing customized inspection and sensing systems for R&D and production. Passionate about advancing quantum sensing applications and integrating novel technologies to meet industry needs.