We are in the midst of the Second Quantum Revolution, a technological shift unlocking new capabilities in quantum sensing, computation, and secure communication. But to understand where we are today, we must first look back at the First Quantum Revolution, which laid the foundation for modern physics.
The First Quantum Revolution: The Birth of Modern Physics
The First Quantum Revolution began in the early 20th century when classical physics failed to explain many fundamental observations, leading scientists to develop the theory of quantum mechanics. This revolution was driven by a series of groundbreaking discoveries that reshaped our understanding of nature at its most fundamental level.
Planck’s Quantum Hypothesis
In 1900, Max Planck introduced the idea that energy is quantized—meaning it comes in discrete packets rather than being continuous. This was formulated through his famous equation:
\[ E = h f \]where:
\(E\) is the energy of a photon.
\(h\) is Planck’s constant:
\[ h = 6.626 \times 10^{-34} \, \text{J} \cdot \text{s} \]\(f\) is the frequency of light.
This discovery explained the blackbody radiation problem, a puzzle that classical physics couldn't resolve. It was the first indication that nature at small scales behaves differently than in our everyday experience.
Einstein’s Photoelectric Effect
Albert Einstein expanded on Planck’s idea by explaining the photoelectric effect, where light striking a metal surface releases electrons. Classical wave theory predicted that any frequency of light should cause electron emission, but experiments showed that only high-energy (short-wavelength) light could do so. Einstein proposed that light behaves as both a wave and a particle, laying the foundation for quantum mechanics.
Schrödinger’s Wave Equation
In 1926, Erwin Schrödinger developed an equation that describes how quantum states evolve over time:
\[ i \hbar \frac{\partial}{\partial t} \Psi = \hat{H} \Psi \]where:
\(\Psi\) is the wavefunction describing the quantum state of a particle.
\(\hbar\) is the reduced Planck’s constant:
\[ \hbar = \frac{h}{2\pi} \]\(\hat{H}\) is the Hamiltonian (energy operator).
The First Quantum Revolution: Impact and Applications
The First Quantum Revolution reshaped the modern world by introducing principles that led to groundbreaking technologies. Scientists did not yet control quantum states directly, but their discoveries allowed the development of devices that fundamentally changed society.
Among the most significant technological outcomes were:
- Semiconductors – Understanding quantum energy levels in materials enabled transistors and microchips, forming the foundation of modern computing and telecommunications.
- Lasers – Controlled emission of photons enabled optical communications, medical treatments, industrial cutting, and scientific research.
- Atomic Clocks – Quantum interactions within atoms allowed for highly precise timekeeping, forming the backbone of GPS technology.
- Magnetic Resonance Imaging (MRI) – The quantum properties of atomic nuclei enabled highly detailed medical imaging.
- Light Sensors and Photodetectors – The photoelectric effect, explained by Einstein, led to innovations in cameras, solar panels, and optical communication.
These advancements shaped industries, from medicine to defense, from telecommunications to computing. But while these devices relied on quantum principles, they did not actively control quantum states.
The Second Quantum Revolution emerged from efforts to manipulate quantum behavior at the individual particle level. It was the precision of lasers, atomic clocks, and highly sensitive sensors—all products of the First Revolution—that enabled researchers to isolate, measure, and control quantum states, paving the way for entirely new applications.
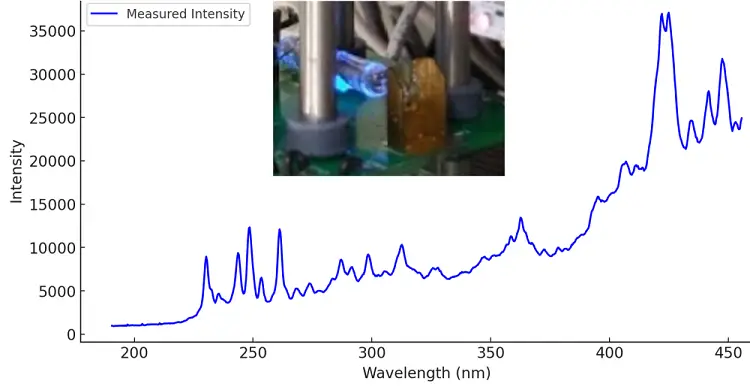
The measured UV spectrum of the Xenon lamp (PXL 100120-00 by IZAK Scientific) exemplifies a fundamental principle of the First Quantum Revolution, where distinct spectral lines correspond to quantized electronic transitions in Xenon atoms, confirming the discrete nature of atomic energy levels and the core principles of quantum mechanics. These sharp emission lines are the foundation of modern photonics applications, including lasers, spectroscopy, and sensors, which drive the Second Quantum Revolution.
The Second Quantum Revolution: A Shift from Observation to Control
The Second Quantum Revolution is fundamentally different because it allows us to engineer and control quantum states, rather than just observing their effects. This shift enables completely new capabilities that classical technologies could not achieve.
Key principles that drive this revolution include:
- Superposition – A quantum system can exist in multiple states simultaneously, leading to powerful computing models.
- Entanglement – Two quantum particles can be correlated, regardless of distance, enabling secure quantum communication.
- Quantum Coherence – The ability to maintain quantum states for longer periods improves precision in sensing and computing.
- Quantum Measurement and Control – New techniques allow information extraction from quantum systems while minimizing collapse.
These principles are now driving transformational quantum technologies that will surpass the limitations of classical physics.
Technologies Emerging from the Second Quantum Revolution
The ability to actively manipulate quantum states has led to innovations in multiple fields:
Quantum Sensing: Ultra-Precision Measurement
Quantum sensors leverage quantum superposition and entanglement to achieve unprecedented accuracy in measuring physical properties. Applications include:
- Magnetometry – Detecting small magnetic fields for medical imaging (quantum MRI), navigation, and fundamental physics research.
- Gravimetry – Mapping underground structures, detecting mineral deposits, and measuring gravitational anomalies.
- Atomic Clocks – Providing next-generation precision timing, critical for satellite navigation, secure communications, and fundamental research.
- Quantum Radar – Enhancing detection capabilities beyond classical radar, useful for defense and autonomous vehicle navigation.
Quantum Computing: A New Model of Computation
Unlike classical computers that process information as binary bits (0 or 1), quantum computers use qubits, which can exist in multiple states simultaneously. This leads to:
- Exponential speedups in solving problems like optimization, cryptography, and material simulations.
- Shor’s Algorithm, which could break classical encryption, making post-quantum cryptography essential.
- Quantum machine learning, revolutionizing AI and pattern recognition.
Quantum Communication: Unbreakable Security
Quantum entanglement and quantum key distribution (QKD) enable secure, tamper-proof communications, impacting:
- Quantum-secure networks for government, finance, and corporate data protection.
- Satellite-based quantum communications, as demonstrated by China’s Micius satellite.
- Next-generation cybersecurity, resistant to computational attacks from future quantum computers.
Quantum Imaging: Beyond Classical Limits
Quantum-enhanced imaging can surpass classical resolution limits, improving:
- Medical diagnostics, with higher-precision scans.
- Astronomical observations, enhancing detection of faint cosmic signals.
- Material analysis, allowing nanoscale structural imaging.
How the Second Quantum Revolution Will Shape the Future
Quantum technologies are expected to drive innovation across multiple industries, including:
- Healthcare – Quantum-enhanced MRI and ultra-sensitive biosensors will improve early disease detection.
- Finance – Quantum computing will optimize risk analysis, fraud detection, and portfolio management.
- Artificial Intelligence – Quantum-powered AI will accelerate deep learning and optimization algorithms.
- Materials Science – Quantum simulations will lead to new superconductors, better batteries, and more efficient solar cells.
- National Security – Quantum cryptography will protect classified data, while quantum radar will enhance defense systems.
- Aerospace and Navigation – Quantum sensors will enable precise navigation in GPS-denied environments, crucial for space missions.
- Climate Science – Quantum computing will enhance climate modeling and improve weather prediction accuracy.
These advances are already moving from research into commercialization. Governments and corporations are investing heavily in quantum technology R&D, with major initiatives including:
- The EU Quantum Flagship Program
- The US National Quantum Initiative
- China’s quantum research investments
- Private sector leaders like IBM, Google, Microsoft, and startups such as Rigetti, IonQ, and PsiQuantum
Bridging the Gap: From Research to Real-World Applications
The transition from fundamental research to industry applications is already underway. Advances in quantum control techniques, error correction, and miniaturization are making quantum devices more practical. In the coming years, we will see:
- Commercially available quantum sensors for medical, industrial, and defense applications.
- Widespread use of quantum encryption in secure communications.
- Increased cloud access to quantum computing platforms for businesses and researchers.
These breakthroughs mark the beginning of a new technological era, one that will fundamentally reshape industries and economies worldwide.
These advancements are no longer just theoretical concepts; they are already shaping industries and leading to real-world applications. As quantum technologies transition from research labs to practical solutions, companies and research institutions are working to bring these innovations to the forefront.
At IZAK Scientific, we specialize in custom quantum sensing solutions, helping industries leverage the precision and power of quantum technology.
We provide expertise in:
- Quantum-enhanced measurement and sensing
- Custom-designed quantum sensor solutions for industrial applications
- Consulting and development for quantum-enabled technologies
Join us at OASIS 2025 (March 10-11) to discuss how quantum sensing can transform your industry.
Follow us for more insights into the Second Quantum Revolution.
References & Further Reading
-
Planck, M. (1901). On the Law of Distribution of Energy in the Normal Spectrum. Annalen der Physik, 4(3), 553-563.
- Introduced the concept of energy quantization, leading to the birth of quantum mechanics.
-
Einstein, A. (1905). On a Heuristic Viewpoint Concerning the Production and Transformation of Light. Annalen der Physik, 17(6), 132-148.
- Explained the photoelectric effect, demonstrating the particle nature of light and laying the foundation for quantum theory.
-
de Broglie, L. (1924). Recherches sur la théorie des quanta [Research on Quantum Theory]. Thesis, Paris.
- Proposed wave-particle duality, showing that matter exhibits wave-like properties.
-
Schrödinger, E. (1926). Quantisierung als Eigenwertproblem [Quantization as an Eigenvalue Problem]. Annalen der Physik, 79, 361-376.
- Formulated the Schrödinger equation, describing how quantum states evolve over time.
-
Heisenberg, W. (1927). Über den anschaulichen Inhalt der quantentheoretischen Kinematik und Mechanik [On the Physical Content of Quantum Kinematics and Mechanics]. Zeitschrift für Physik, 43, 172-198.
- Introduced the uncertainty principle, defining fundamental limits to measurement in quantum mechanics.
-
Nielsen, M. A., & Chuang, I. L. (2010). Quantum Computation and Quantum Information (10th Anniversary Edition). Cambridge University Press.
- A comprehensive introduction to quantum computing, quantum algorithms, and quantum information theory.
-
Dowling, J. P., & Milburn, G. J. (2003). Quantum Technology: The Second Quantum Revolution. Philosophical Transactions of the Royal Society A: Mathematical, Physical and Engineering Sciences, 361(1809), 1655-1674.
- Discusses the transition from the First to the Second Quantum Revolution and the emerging applications of quantum technology.
-
Acín, A., Bloch, I., Buhrman, H., et al. (2018). The Quantum Technologies Roadmap: A European Perspective. New Journal of Physics, 20(8), 080201.
- A roadmap for quantum technologies, including quantum sensing, computation, and communication.
Tzachi Sabati
CEO, IZAK Scientific
Physicist specializing in photonics and quantum technologies, with deep expertise in quantum sensors and advanced optical systems. Leads the Advanced Quantum Lab course at the Technion, bridging academic excellence with industry innovation. At IZAK Scientific, provides cutting-edge photonics-based solutions, developing customized inspection and sensing systems for R&D and production. Passionate about advancing quantum sensing applications and integrating novel technologies to meet industry needs.