Introduction:
Photon entanglement, a phenomenon straight out of the quantum realm, has long fascinated scientists and laypeople alike. This enigmatic feature of quantum mechanics, where pairs or groups of photons exhibit a connectedness that transcends the bounds of classical physics, is not just a theoretical curiosity. It holds the potential to revolutionize various fields, from computing to communication and beyond.
The realm of quantum physics brings us to the fascinating concept of photon entanglement, a phenomenon that defies the very fabric of traditional physics and has potential implications far beyond our current understanding. This phenomenon, where photons are interconnected in such a way that the state of one instantly influences its partner, irrespective of the distance, is not just a theoretical marvel but a practical tool poised to revolutionize multiple sectors. In this blog, we delve deeper into photon entanglement and its diverse applications.
Understanding Photon Entanglement:
Photon entanglement occurs when pairs or groups of photons are generated in a state that intertwines their properties, such as polarization or phase. The entangled photons maintain a quantum connection that Einstein famously referred to as “spooky action at a distance.” The peculiar aspect of entangled photons is their ability to influence each other instantaneously over large distances, challenging our conventional notions of space and time.
Revolutionary Applications of Photon Entanglement:
Quantum Computing:
Quantum computers leverage the principles of entanglement to perform complex computations at speeds unattainable by traditional computers. Entangled photons can exist in multiple states simultaneously, allowing quantum computers to process a vast amount of data more efficiently.
Quantum computing stands as one of the most exciting applications of photon entanglement. In quantum computers, entangled photons enable simultaneous processing of a multitude of possible outcomes, offering exponential speed increases over traditional computers. This capability makes quantum computers exceptionally powerful for tasks like database searching, cryptography, and simulation of quantum phenomena.
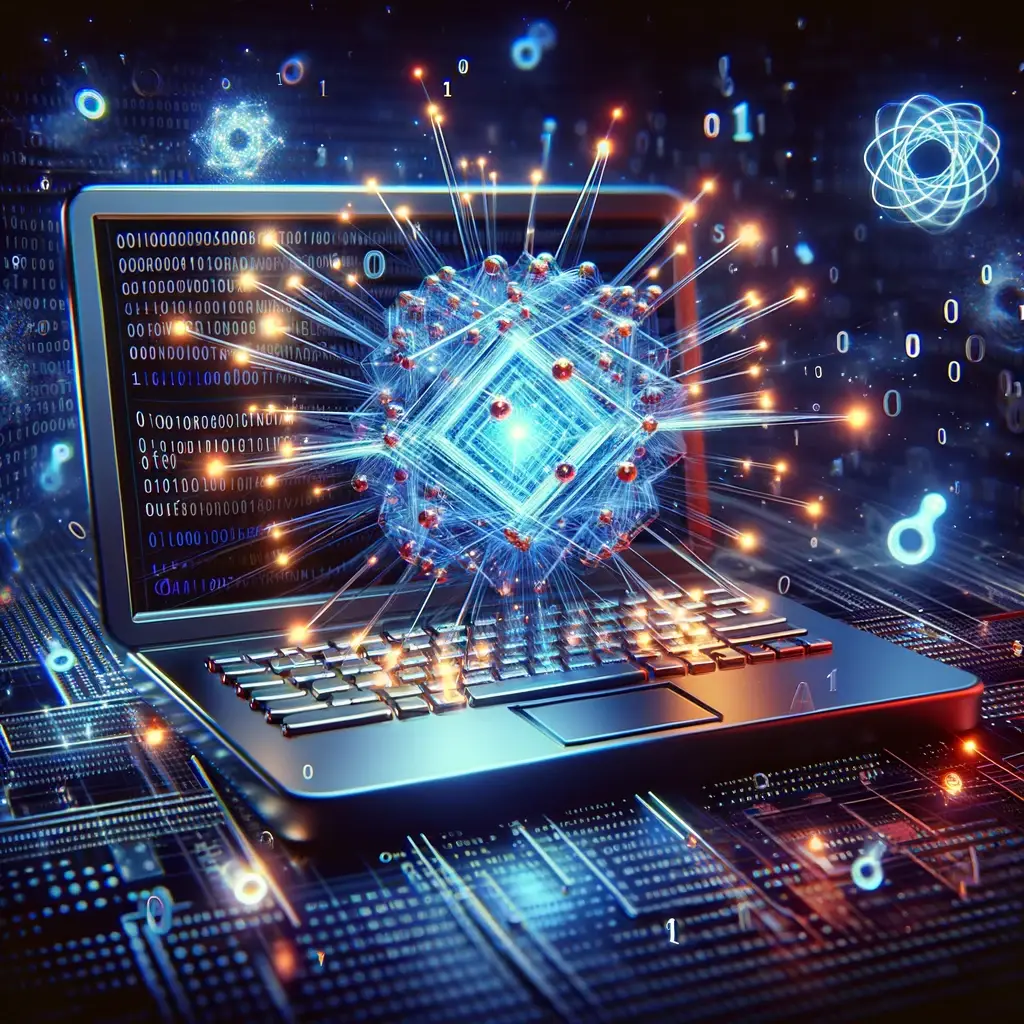
Quantum Cryptography:
The security of information transmission is a paramount concern in the digital age. Quantum cryptography uses entangled photons to create unbreakable encryption keys. Any attempt at eavesdropping changes the state of the entangled photons, alerting the sender and receiver to a breach.
Quantum cryptography represents a leap in secure communication. It employs entangled photons to generate cryptographic keys that are theoretically unbreakable. Any eavesdropping attempt on the quantum key distribution (QKD) system disturbs the entangled state, making the intrusion detectable. This technology promises a new era of secure communication against the backdrop of increasing cyber threats.
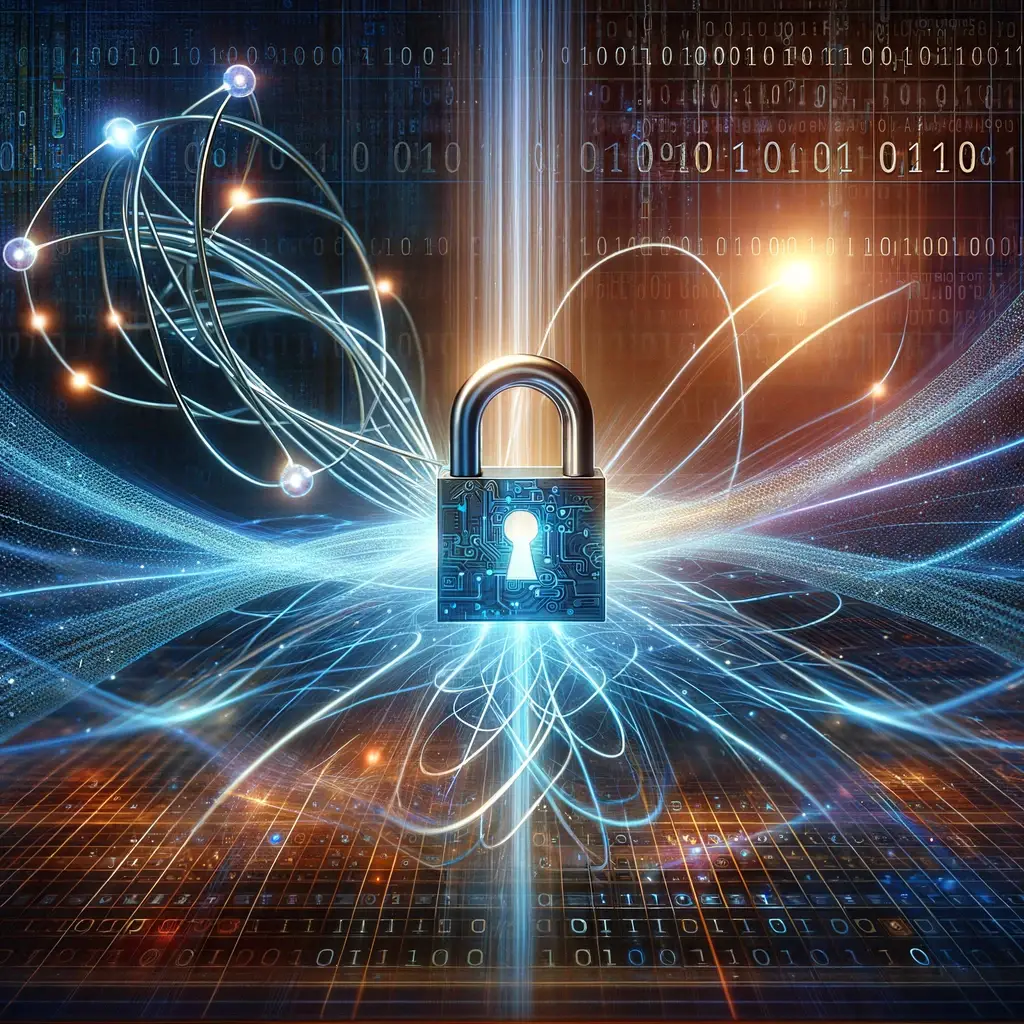
Quantum Imaging and Sensing:
Quantum imaging techniques, harnessing the power of entangled photons, can capture images with unparalleled resolution and sensitivity. This has profound implications for medical imaging and astronomical observations, where detecting faint light signals is often crucial.
Quantum imaging utilizes entangled photons to surpass the limitations of classical imaging. It enables capturing images with unprecedented precision, useful in scenarios where traditional imaging fails, such as observing biological processes without damaging tissues or detecting faint astronomical objects.
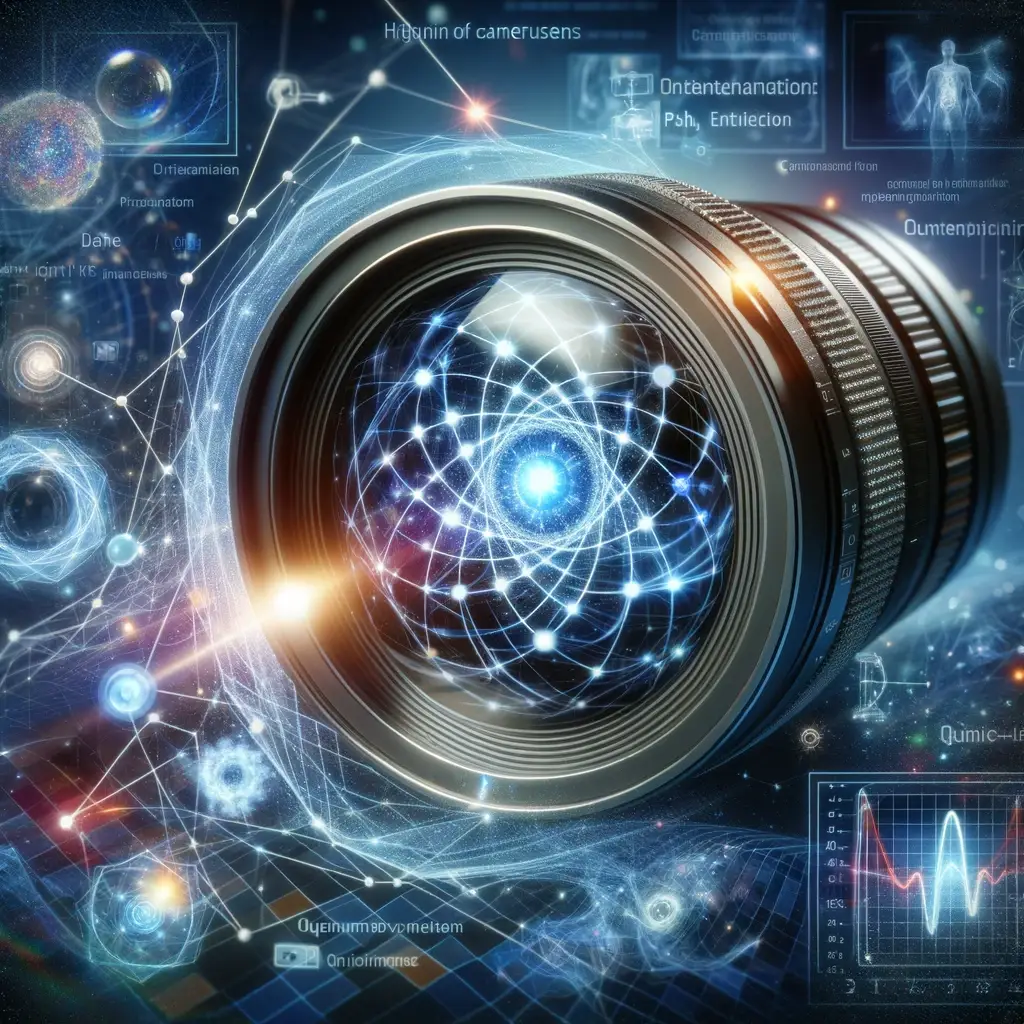
Quantum Teleportation:
While it may sound like science fiction, quantum teleportation is a reality, albeit not in the way popular culture imagines it. Researchers have successfully teleported the quantum state of a photon to another photon at a different location, paving the way for secure communication channels and a quantum internet.
Quantum teleportation, achieved through photon entanglement, involves transmitting the quantum state of a particle to another particle located at a distance. While it doesn’t involve physical transportation, it’s crucial for realizing long-distance quantum communication and intricate quantum networks.
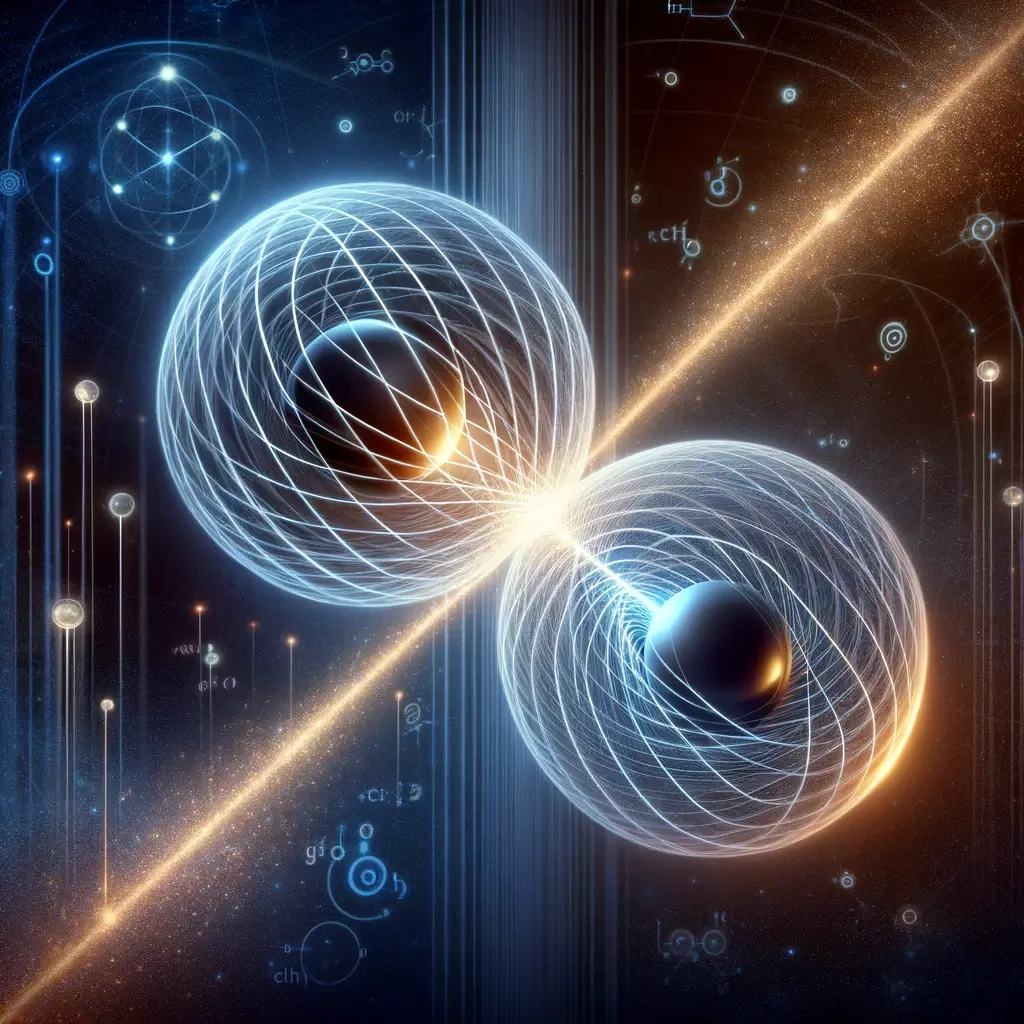
Challenges and the Future:
While the potential of photon entanglement is immense, there are technological and scientific challenges in harnessing its full potential. Issues such as maintaining entanglement over long distances and developing scalable quantum systems for practical applications are areas of active research. However, the progress made so far is a strong indicator of the transformative future that lies ahead with photon entanglement, the strides made in recent years are promising.
Conclusion:
Photon entanglement, once a perplexing quantum oddity, is now at the forefront of cutting-edge technology. From bolstering cybersecurity to redefining computing, its applications are as profound as they are varied. As we continue to delve deeper into the quantum world, the full scope of photon entanglement’s impact on our future remains an exciting, unfolding mystery.
Photon entanglement, with its inherent quantum weirdness, stands not just as a testament to our scientific advancements but as a beacon guiding us towards a future replete with technological marvels once thought impossible.
Photon entanglement, a cornerstone of quantum physics, is steering us towards a future where quantum computing, secure communication, advanced imaging, and a deeper understanding of the universe could become everyday realities. As research and technology evolve, we may soon witness the full extent of its impact on our world.
References:
1. Einstein, A., Podolsky, B., & Rosen, N. (1935). Can Quantum-Mechanical Description of Physical Reality Be Considered Complete?
2. Bennett, C. H., & Brassard, G. (2014). Quantum Cryptography: Public Key Distribution and Coin Tossing.
3. Bouwmeester, D., Pan, J. W., Mattle, K., Eibl, M., Weinfurter, H., & Zeilinger, A. (1997). Experimental Quantum Teleportation.
4. Giovannetti, V., Lloyd, S., & Maccone, L. (2004). Quantum-Enhanced Measurements: Beating the Standard Quantum Limit.
5. Aspect, A., Grangier, P., & Roger, G. (1982). Experimental Realization of Einstein-Podolsky-Rosen-Bohm Gedankenexperiment: A New Violation of Bell’s Inequalities.
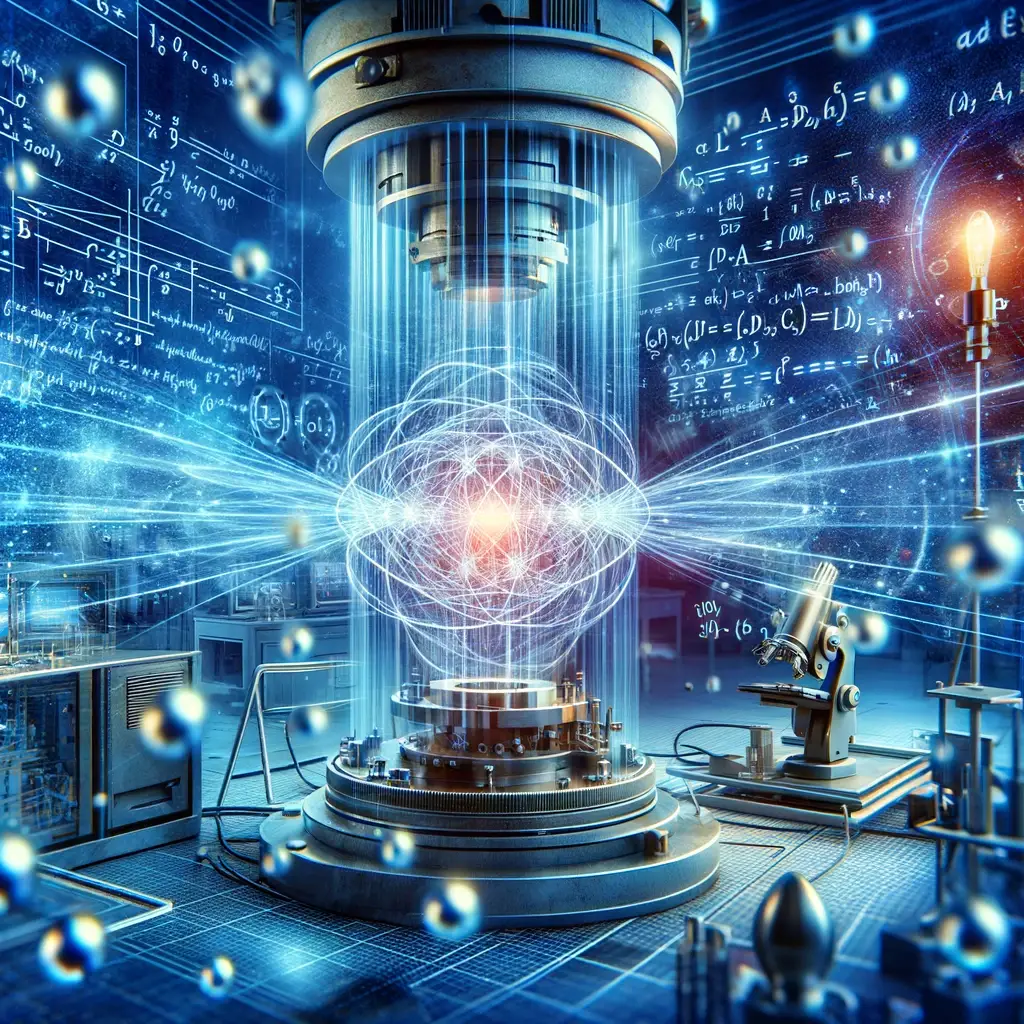
Tzachi Sabati
CEO, IZAK Scientific
Physicist specializing in photonics and quantum technologies, with deep expertise in quantum sensors and advanced optical systems. Leads the Advanced Quantum Lab course at the Technion, bridging academic excellence with industry innovation. At IZAK Scientific, provides cutting-edge photonics-based solutions, developing customized inspection and sensing systems for R&D and production. Passionate about advancing quantum sensing applications and integrating novel technologies to meet industry needs.